Frequent charging of the high-voltage battery of electric vehicles (EVs) translates to high mechanical stress requirements for charging cables and connectors. If the insulation breaks and live metal parts are exposed, or shunts appear in the onboard electronics, life-threatening residual currents can occur in the EV user’s body. Particularly problematic are various DC residual current forms that cannot be detected by the AC-sensitive Type-A residual current devices (RCDs).
To prevent electric shock accidents, manufacturers of EV supply equipment (EVSE) must incorporate RCDs into their power electronics products that trip within a few milliseconds for both AC and DC residual currents of a few milliamperes (mA).
This article explains forms of residual currents, how to measure them, and where to install the RCD in the charging circuit. It then introduces residual current monitors (RCMs) from Littelfuse that system designers can use to add protection against DC electric shock in their EVSE devices in a cost and time-efficient manner. The article also demonstrates which EV charging modes these current sensors are suitable for and how they are used.
Residual currents in the EV charging circuit
Charging EVs at voltages up to 400 volts AC and 1000 volts DC requires extensive protective measures for the EV user when handling charging equipment. Due to the harmonic-rich and asymmetric switching pulses of charging stations and onboard chargers, as well as several hundred volts of DC link voltage, various types of AC and DC residual currents can occur via shunts, coupling effects, insulation faults, and leakage faults.
Power electronic circuits such as rectifiers, switching converters, frequency inverters, along with inverter and phase-angle control systems, have a wide variety of load current characteristics. The resulting potential residual currents are categorized as sinusoidal AC, pulsed DC, and straight DC. These residual current forms are dangerous to humans. Table 1 shows typical load current signals of various circuit topologies and the resulting residual current waveforms. Columns 1 to 3 assign RCD types suitable for detection.
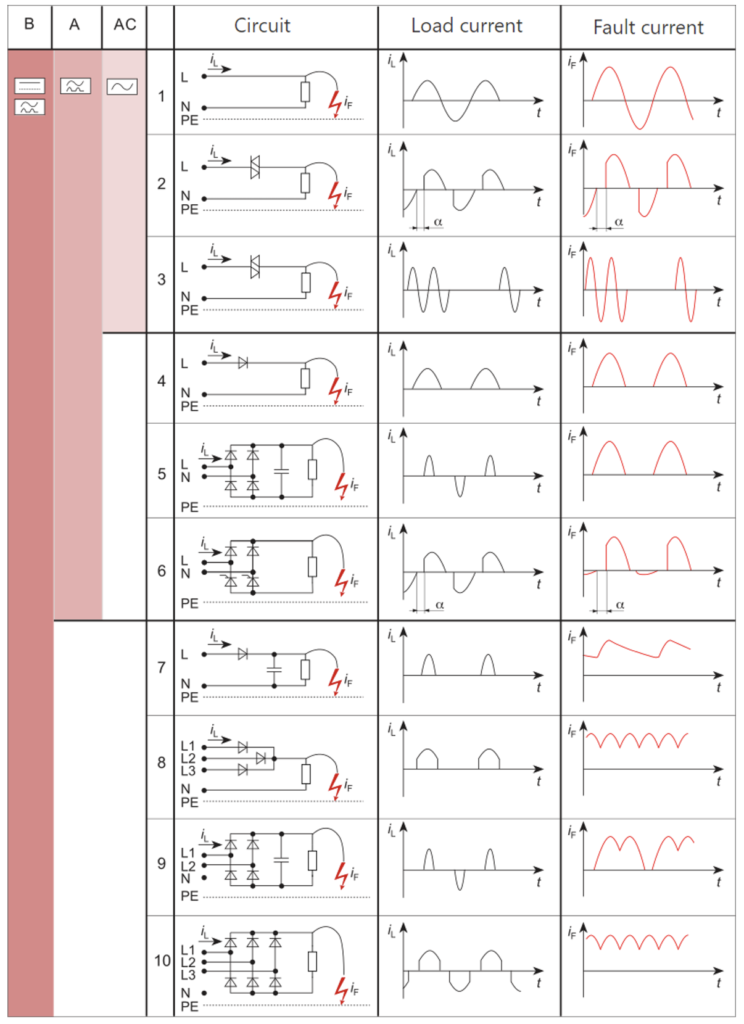
A good knowledge of residual current waveforms can help EV repair shops and electricians when tracking down residual currents in EV board electronics, EVSE, or charging stations.
Tripping characteristic of RCD types
In general, personal protection against electric shock on electrical installations is regulated by IEC 60479 and UL 943. Both standards define significant AC and DC residual currents in the range of 6, 30, 100, 300, 500, and 1000 mA, at tripping times ranging from 20 to 500 ms. Common tripping thresholds in the EV charging circuit are 6 mA DC and 30 mA AC.
System designers can now easily implement specific personal protection requirements in the charging circuit by selecting an RCD type of the appropriate standard. Table 2 lists residual current forms and trip tolerance of the different RCD or ground fault circuit interrupter (GFCI) types.
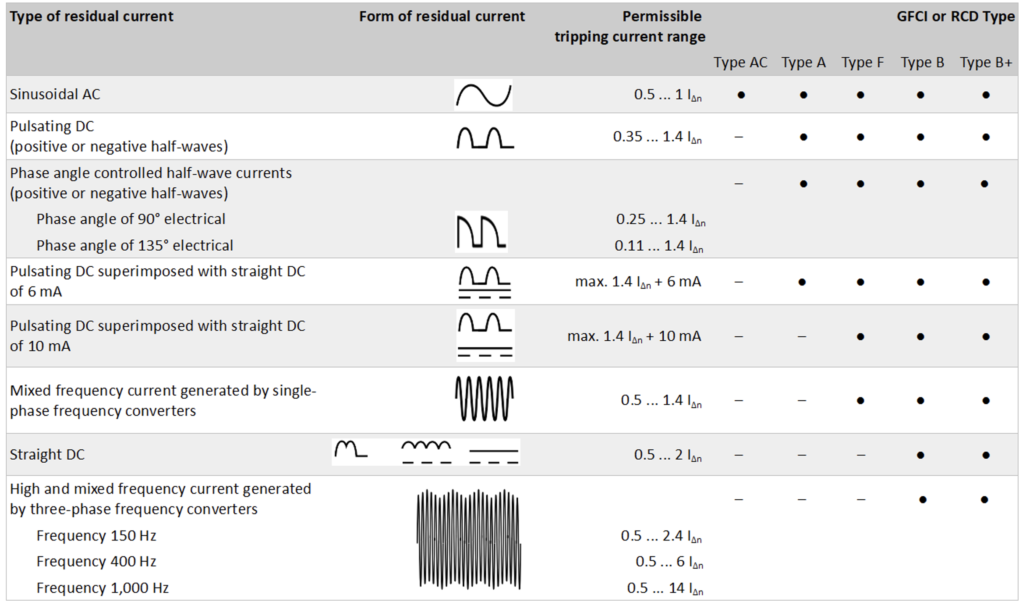
Installing RCDs in the EV charging circuit
Type-A or Type-F RCDs only detect AC residual current and DC pulsating current, which is insufficient for protecting an EV charging circuit. A wide range of straight DC residual currents that can occur in the onboard charger or battery management system must also be considered.
The IEC 62196 standard, therefore, defines two residual current protection options: the use of an all-current sensitive RCD of Type B (or Type B+), or an RCD of Type A in conjunction with a residual DC monitoring system according to IEC 62955 with IΔn DC ≥ 6 mA. The DC fault current monitoring can be arranged in the wall box, in the building electrical installation, or at both locations.
Since an AC-sensitive Type-A or Type-F RCD is usually present in building electrical systems, designers can cost-effectively add 6 mA residual DC monitoring to Mode 3 wall boxes or charging stations, as well as to the in-cable control boxes (ICCB) of Mode 2 charging cables (Figure 1, cases 2 and 3).
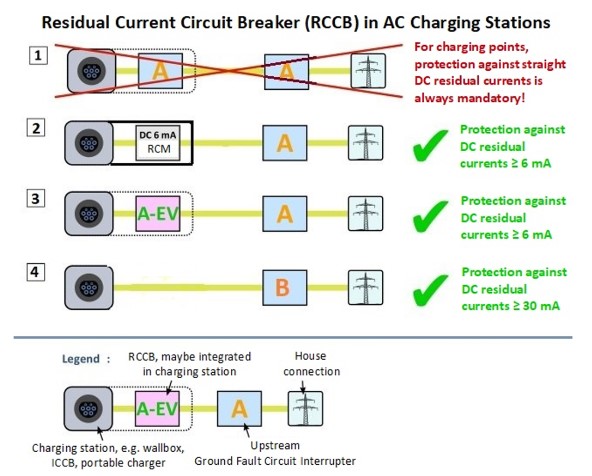
Charging modes for EVs
The EV battery can be charged via different charging modes, depending on the available on-site power connection, connection plug, charging cable, and the charging technology installed in the vehicle, as well as in the charging station. In Europe, the electrical energy can be fed into the vehicle via single-phase AC (230 volt/3.6 kilowatt (kW), three-phase AC (400 volts/22 kW), or via high-voltage DC charging stations (up to 1000 volts DC/500 kW). Figure 2 illustrates the four charging modes defined in the IEC 61851 standard.
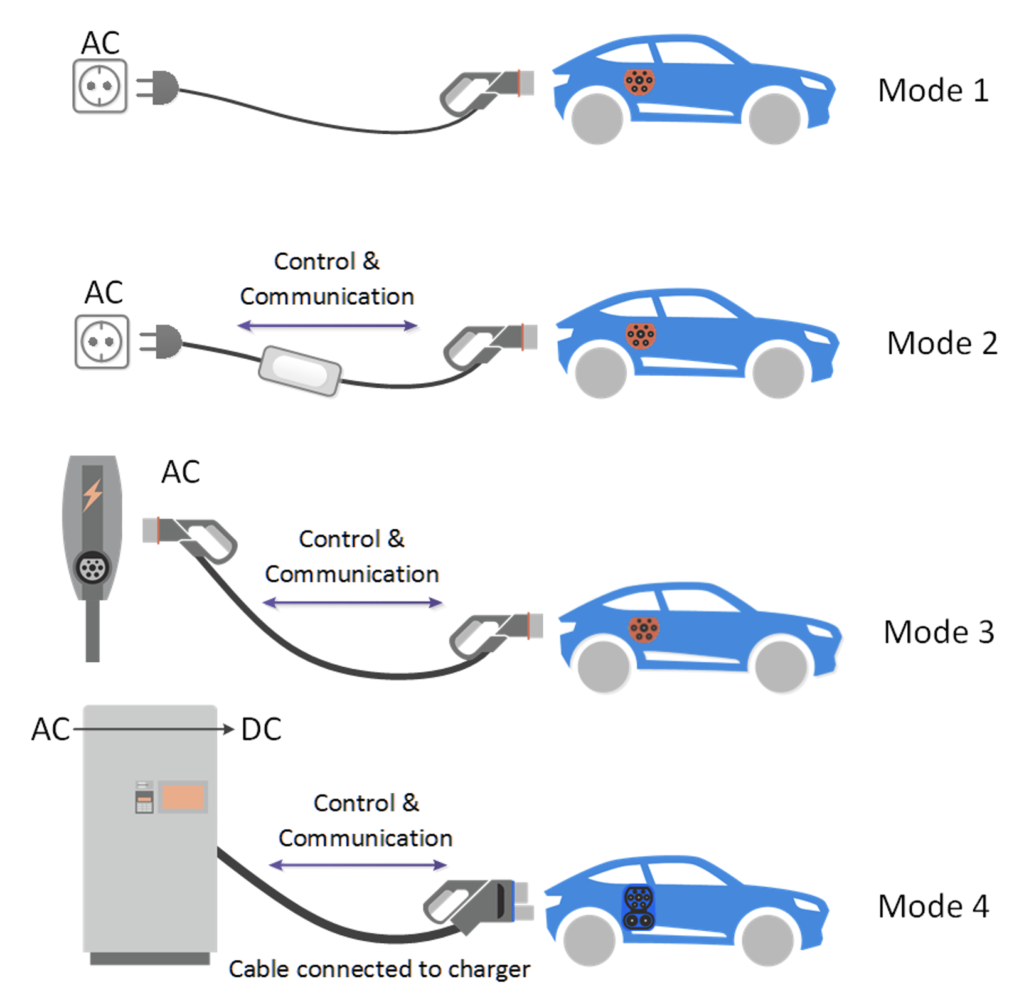
(Image source: bestchargers.eu)
Mode 1 (single-phase AC charging up to 3.6 kW; default mode of charging)
In this case, the electric or hybrid vehicle is connected to a standard 230 volt household socket using a simple passive cable, and will be charged using low power at a maximum of 3.6 kW via the onboard charger. This charging scenario does not provide sufficient protection against residual DC for the user. Usually, only an AC-sensitive Type-A RCD is installed in the building’s electrical system.
Mode 2 (single/three-phase AC charging up to 22 kW via an ICCB charging cable)
A Mode 2 charging cable equipped with a Type 2 vehicle plug contains an ICCB that performs safety and communication functions when charging EVs using domestic and three-phase sockets to prevent overloading them.
The following protection functions must be integrated with the ICCB:
- Determination of polarity and protective conductor (PC) monitoring; only a few ohms of loop impedance are permitted between neutral and the PC.
- Testing of the electrical connection between the PC and the metal body.
- An AC and DC residual current circuit breaker prevents current accidents.
- Monitoring/shutdown of the charging process in the event of anomalies (for example, current fluctuations due to corroded plug contacts or cable breakage).
- Monitoring of the temperature inside the ICCB and both plugs, and perform shutdown if necessary.
- Control of the charging power: Pull-down resistors on the control pilot (CP) wire to signal the cable current load rating to both the wall box and the EV; the pulse width modulation (PWM) signal on the charging control (CC) wire signals the wall box charging power capability to the EV.
Mode 3 (single-phase/three-phase AC charging up to 22 kW via wall box)
For EV charging, a passive Mode 3 cable is connected to a wall box in private households or a public AC charging station in parking lots. Both have integrated the same protection functions as the ICCB above.
Mode 4 (direct battery DC fast charging up to 500 kW)
DC high-power charger (DC/HPC) stations for EVs deliver significantly higher charging currents compared to Mode 2 and Mode 3. Shock protection from residual AC and DC is implemented in this supercharger; the different charging cables are always firmly attached.
Measure AC and DC fault currents in the EVSE circuit
RCMs, from the RCM14 series from Littelfuse Inc., detect DC and/or AC residual currents in AC or DC systems and deliver an output signal to control an external disconnect (cutoff relay). In contrast, RCDs and residual current circuit breakers (RCCBs) have an integrated cutoff relay.
AC residual currents are detected using an inductive current transformer (CT). For this purpose, the current forward conductor (IL) and current return conductor (IN) are fed through a soft magnetic toroidal core, causing both current vectors to normally compensate for each other and add up to zero. If a fault current (Ig) flows into the ground potential via the human body in the circuit behind the detector, the RCM or GFCI total current differs from zero, and the circuit breaker trips (Figure 3).
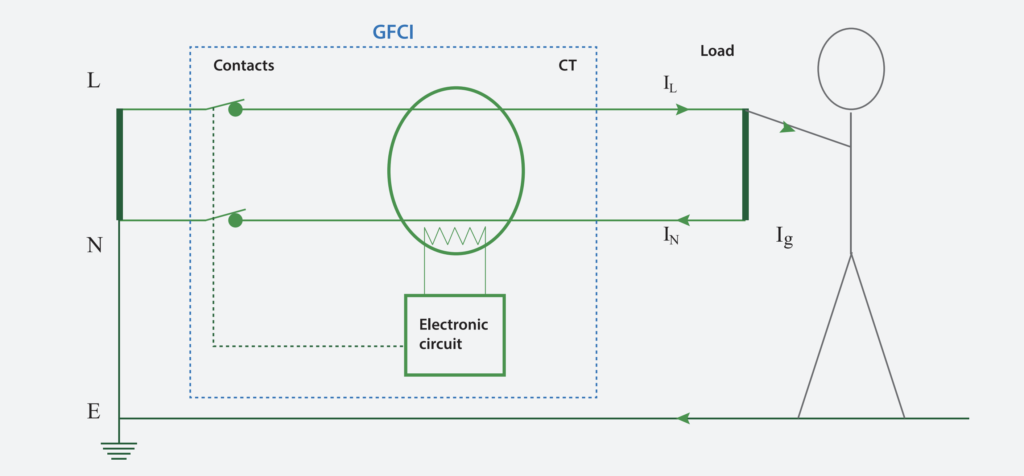
By integrating a fluxgate magnetometer probe into a slot of the toroidal core and compensating the magnetic flux to zero by means of a compensation coil, the CT can also detect differential DC. More accurate than Hall effect sensors or shunt resistors, this method detects tiny DC fault currents from 6 mA at heavy DC load currents up to 500 amperes (A).
RCMs featuring control output for disconnector
Littelfuse’s RCM14 series is ideal for use in ICCB charging cables for EVs (Mode 2) and EV charging stations (Mode 3). They are available in three residual current detection options in accordance with IEC 62752 (Mode 2), IEC 62752 (Mode 3), and UL 2231.
Each RCM has one operation LED and one fault LED. The four-pin JST connector simplifies the installation: pins 1 and 2 are for the 12 volt power supply, pin 3 is for external function testing, and pin 4 is an open-drain switching output to drive an external disconnector such as a cutoff relay, at up to 100 mA and 24 volts (maximum) (Figure 4).
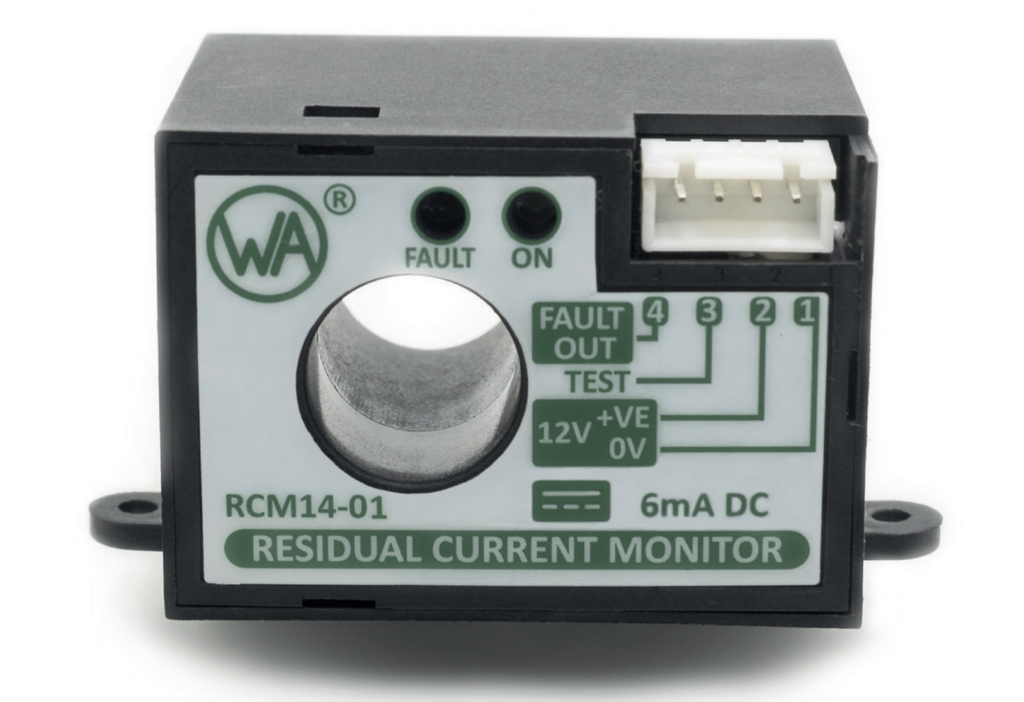
These active RCMs can also be used to detect AC and/or DC residual currents in single-phase or multi-phase DC installations. Single-phase operation limits the load current to 100 A, while three-phase operation is at 40 A. They can handle load current pulses up to 3000 A.
RCM14-01: 6 mA DC RCM module according to IEC 62955, 14 millimeter (mm) aperture
The RCM14-01 residual current monitor detects DC fault currents in 50 Hz/60 Hz AC systems. It is developed for use in Mode 3 charging stations for EVs (IEC 62955 standard) to interrupt the charging circuit of the EV in the event of a DC fault current ≥ 6 mA. This detector adds DC residual current monitoring function to existing Type-A and Type-F RCDs of the building’s electrical system in a cost-effective and simple manner (Figure 5).
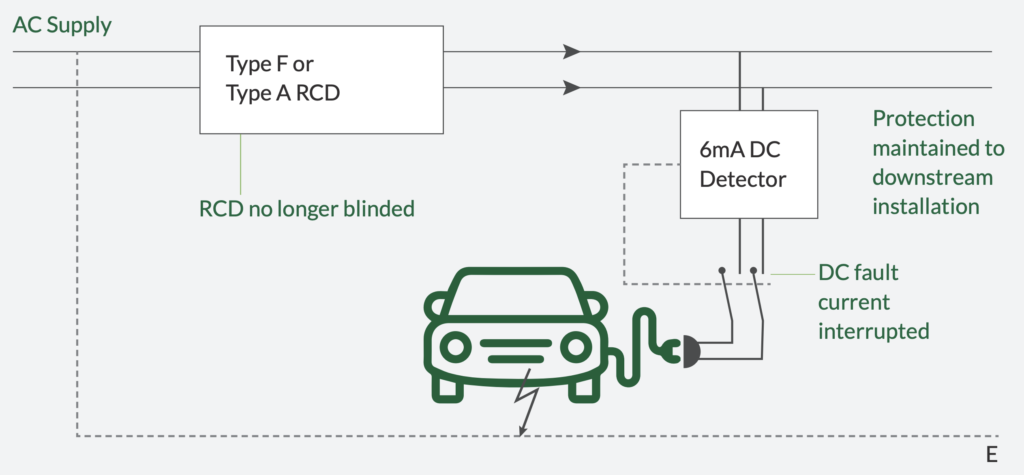
RCM14-03: 6 mA DC/30 mA AC RCM module according to IEC 62752, 14 mm aperture
The RCM14-03 is intended for use in ICCB or integrated protection devices for EVs in charging Mode 2 to interrupt the supply to the EV in the event of an AC or DC fault.
RCM14-04: 56 mA DC/20 mA AC RCM module according to UL 2231-2, 14 mm aperture
The RCM14-04 module detects AC and DC fault currents in 60 hertz (Hz) AC installations. It is designed for use in Charging Circuit Interrupting Device (CCID) EV charging station applications where it interrupts the supply to the EV in the event of an AC and/or DC residual current condition.
For integration in a larger device circuit, the following RCM modules presented are also available as open-frame systems:
- RCM14-01_SYS_V, RCM14-01_SYS_H
- RCM14-03_SYS_V, RCM14-03_SYS_H
- RCM14-04_SYS
Each system consists of a solderable sensor pc board and a separate current transformer (Figure 6).
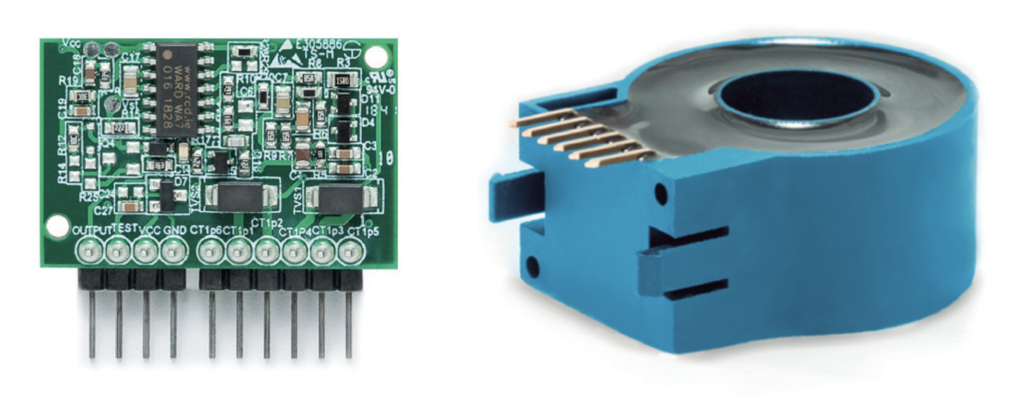
Conclusion
AC-sensitive Type-A RCDs are common installation standards in building electrical systems, but they cannot protect against DC residual current hazards in EV charging circuits. As shown, the RCM14 series can perform the DC residual current monitoring required in ICCB charging cables (Mode 2) and EV charging stations (Mode 3). Featuring only four connection pins, system designers can easily and cost-effectively implement the compact RCM module or the open-frame system in their EVSE.