Power semiconductors are tiny electronic devices that regulate and convert electrical power. Power semiconductors, in contrast to basic semiconductor devices, can sustain high voltage and current with reduced leakage, voltage drop, and other power losses. The current and voltage we deal with in power semiconductors are measured in kilos and megavolts. They are utilised in a wide range of applications, from the power supply and motor control to lighting control and renewable energy systems.
In this cover story, we will explore the world of power semiconductors, from their humble beginnings to their present-day role as critical enablers of a sustainable future.
Early Days:
Vacuum tubes were the mainstay of electronic devices until the introduction of power semiconductors. They were used in early radio and television receivers, as well as in early electronic computers. Vacuum tubes were also used in early power supplies, where they were used to rectify AC power into DC power.
Solid-state diodes soon replaced vacuum tubes thus bringing a revolution in electronic devices. These diodes were smaller, more reliable, and more efficient. Solid-state diodes were also used to rectify AC power into DC power, but they were much more efficient than vacuum tubes. In the 1960s, the first solid-state power transistors were developed. These transistors were used in power amplifiers and switching applications.
The Rise:
In the 1970s and 1980s, power electronics began to emerge as a new field of study. The field included the application of electronics to the control and conversion of electrical power, the design of power semiconductors, as well as the design of circuits and systems that use power semiconductors.
One of the key drivers of power electronics was the need for more efficient power conversion. Traditional electromechanical switches, such as relays and contactors, were relatively slow and inefficient. Power semiconductors, on the other hand, were much faster and more efficient.
Another key driver of power electronics was the rise of renewable energy. Renewable energy systems, such as solar and wind power, produce DC power that must be converted into AC power for use on the grid. Power electronics are used to convert DC power into AC power.
Types of Power Semiconductors:
There are several different types of power semiconductors, each with unique characteristics and applications. Some of the most common types of power semiconductors include:
Power Diodes: Power diodes are used to convert AC power into DC power. They are also used in voltage regulators, where they are used to maintain a constant voltage.
Power Transistors: Power transistors are used in power amplifiers and switching applications. They can handle high levels of voltage and current, and they are very fast.
Insulated Gate Bipolar Transistors (IGBTs): IGBTs are used in high-power applications, such as motor drives, power supplies, and renewable energy systems. They are very fast and can handle high levels of voltage and current.
Metal Oxide Semiconductor Field-Effect Transistors (MOSFETs): MOSFETs are used in power electronics, such as DC-DC converters.
Challenges with Silicon:
In general, efficiency is mainly limited by the performance of semiconductor devices used in power conditioning and conversion systems. Silicon (Si) is currently the most used semiconductor for power devices. Si is abundant, and large-high-quality single crystals can be produced at an economical cost, which makes it dominant in the electronics industry, including power devices. The performance of Si power-switching devices has been significantly improved in the last 3 decades through the development of power metal oxide semiconductor field-effect transistors (MOSFETs) and insulated gate bipolar transistors (IGBTs). However, in recent years this prominent material has faced limitations in certain application fields because of its intrinsic material properties; one of these fields is high-blocking voltage (>600 V) power device applications. Now that Si power device technology is relatively mature, it is not easy to achieve innovative breakthroughs in power semiconductor devices using this material, and thus there are high hopes for the emergence of novel power semiconductor materials, such as silicon carbide (SiC) and gallium nitride (GaN).
Innovation:
The technological potential of Silicon Carbide (SiC) for power devices stems from its outstanding combination of physical and electronic properties. The large bandgap (roughly three times that of Si) enables device operation at increased temperatures (873 K for transistors) and low-leakage currents. SiC can withstand high electric fields before the breakdown, 10 times greater than Si, and also high current densities. The large electron saturation drift velocity enables SiC to generate high power at high frequencies. SiC has excellent thermal conductivity, greater than copper at room temperature, which makes it suitable for high-power operation. Its strong chemical stability is a prominent advantage for sensors intended to operate in severe environments, for example in the aerospace and automotive industries.
Challenge:
However, around 1973 many institutes halted research when they failed to grow SiC in large single crystals. In 1978 Tairov and Tsvetkov made a major step in the development of SiC, with the use of a physical vapor transport (PVT) growth technique, the so-called “modified-Lely method,” for SiC bulk crystal growth, and this breakthrough led to the possibility of growing large single crystalline SiC. In the last decade, SiC bulk crystal growth technology has achieved significant progress and enabled the growth of large-high-quality SiC crystals.
The first commercial SiC power devices were Schottky barrier diodes, released by Infineon Technologies, Germany, in 2001. Today more than 20 device manufacturers produce SiC Schottky barrier-based diodes with a breakdown voltage higher than 600 V. SiC power MOSFETs are also available in the market, but they still have problems related to manufacturing costs and reliability, and these problems largely stem from the SiC crystals used for device manufacturing. Thus, low-cost manufacturing of large-size, high-quality SiC crystals is required to enable SiC power devices to penetrate the market further and enhance their adaptation to a wide range of power electronic systems.
Another important requirement for SiC crystal growth is low densities of defects. In the last 2 decades, significant progress in defect reduction has been made, and now low-defect-density SiC substrates are commercially available for manufacturing SiC power devices.
Gallium Nitrate:
Gallium Nitrate (GaN) is the second most important material after Silicon. It is also a wide band gap material i.e 3.49 eV which is more than 3.25 eV of Silicon Carbide. GaN is a good conductor of heat and has high heat and voltage tolerance. Its industrial use began with optoelectronics like LEDs, Lasers, displays, and lights.
Due to GaN HEMT’s inherent ability to move more electrons, dyes can be made much smaller. For smaller voltages up to 650 V or so, GaN components can be made denser and can handle high switching frequencies. So, more power is in a smaller package, making it more suitable than SiC. It is currently being used in high-power mobile phone chargers and EV mobility. Further, it has potential applications in data centers where DC power networks are being deployed to lower conversion losses. This requires new DC-DC systems where GaN can play a significant role.
Challenge:
It is not easy to make a pure crystal of Gallium Nitrate. Homoepitaxy is difficult because it has a high melting point of 2000oC and pressure of 60000 bar which means creating melt to create crystal is difficult and hetroepitaxy is economically unviable as the substrate Sapphire, currently being used, is rare and costly.
GaN technology is still in the early stage and continues to grow.
SiC and GaN:
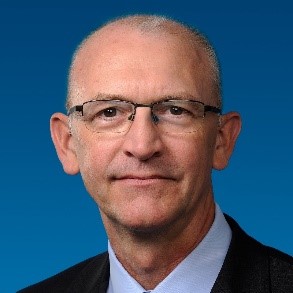
On being asked, how are SiC and GaN semiconductors different and whether GaN can be seen as a replacement for SiC? Doug Bailey, VP Marketing at Power Integrations said, “GaN is a lateral technology and so it is most likely to serve low current applications first, before moving later to high currents. I’d expect applications requiring power levels of less than 10 kW (say 10A with 50A peak) – including on-board chargers (OBC) in EVs, motor drives, air conditioning units and bus converters in large industrial servers – to move to GaN quickly and then for GaN to expand into higher power applications as it matures.”
“SiC will likely be squeezed in the middle between the ‘best’ and the ‘cheapest’, which in high tech can be a difficult position to occupy. Raw elemental availability for the materials making up both GaN and SiC is relatively unconstrained and so the difference in cost will eventually settle down to the huge amount of energy that processing SiC demands. As a vertical technology, SiC devices tend to have a lower specific RDS(ON) than the lateral GaN, so SiC is likely to be advantageous for high-current applications. But fundamentally, if GaN can satisfy the requirement – and increasingly it can – it should be the logical choice,” he further adds.
Modern Application:
The increasing demand for renewable energy and electric mobility has increased the demand and innovation in power semiconductors.
Power Si devices are not used over 150 °C, because power loss increases due to an increase of leakage current in the off-state under a high-temperature environment, thereby decreasing their reliability. GaN devices are expected to work over 200 °C and possibly higher, because of their wider bandgap. An example best shared with the present hybrid vehicles having two cooling systems, one is for the engine and the other is for the inverter, and the temperature of coolant water for the inverter is lower than that for the engine. If GaN devices work over 200 °C, the cooling system of hybrid vehicles will be simplified and its cost will be reduced. The same example can be extended to other applications like PV inverters, where heat sink forms the majority of the volume. Higher temperature operation will reduce cooling requirements and cooling costs, effectively shrinking the size and overall system cost.
Companies are innovating new components and a debate has been started around which is the best material for these applications.
Doug said, “In some fundamental respects, mobility markets are the same as others – power requirements are increasing, and the size allocated for hardware is shrinking. Therefore, increased efficiency is required. With electric vehicles, this is even more important, since the performance of EVs – range/speed – is highly dependent on the weight. This is driving intense innovation in power semiconductors and particularly in Wide Bandgap (WBG) technologies such as gallium nitride (Ga) and silicon carbide (SiC), which offer significantly increased efficiency over traditional silicon.”
“Presently, SiC is mainly found in drivetrain applications where its higher voltage capability (1700V) is extremely useful. GaN is mostly found in lower current applications, but is increasingly used at higher voltages too – Power Integrations has just launched a 900V GaN flyback converter power supply IC – InnoSwitch3-AQ – and the industry can easily envisage 1200V devices. GaN power semiconductors are being used in power supplies to replace 12 V lead-acid batteries and in on-board chargers.,” he further added.
Research:
Although Silicon technology is mature enough there is still a lot of room for innovation in the field of Silicon Carbide and Gallium Nitrate. The manufacturing process to create pure crystal, lesser defect density, reliability and overall cost still demand significant improvements.
Further, highlighting on the range of research in the domain of power electronics, Doug said, “Currently there is research into increasing the voltage of GaN devices, which will mean that SiC devices – which are more expensive to manufacture – will be less attractive. Power Integrations now has a 900V GaN device. At Power Integrations we are technology agnostic: we use whichever technology is best for the application; traditional silicon at low powers and for well-behaved mains voltages, our POWIGaN up to 900V and SiC for its superb voltage withstand performance and efficiency.”
In conclusion, we have come a long way since vacuum tubes. Modern requirements have further enabled research in new materials and specifications for power electronics. Silicon Carbide and Gallium Nitrate offer many advantages which are very basic for demanding applications these days. These materials are the future of power electronics but there are still miles to go.